To Search All Text On This Page
On Windows – Press Ctl+F
On Mac – Command+F
This site is suitable for a wide range of users, with technical information
levels 1 and 2 available on closed toggles
Technical level 1 Technical level 2
Fire development and fire load
- Typical stages of fire development
Fire severity
- Nominal temperature-time curves
- Parametric fire curves
Fire safety design
- Fire safety objectives
- Occupancy categories
- Means of fire protection and evacuation safety
-
- Passive fire protection
- Active fire protection
- Evacuation safety
- Means of fulfilling fire safety objectives
-
- Prescriptive codes
- Performance-based codes and design tools
- Statistical analysis of fires in timber structures
-
- Further findings
Fire development and fire load
Once ignition occurs, the fire can develop and behave in many ways, growing very rapidly, very slowly (as a smouldering fire) or even self-extinguishing, depending on the arrangement of combustible materials in the vicinity of the ignition source, on the type and amount of combustible material, and on the geometry, dimensions and ventilation of the room.
For the purposes of design, a typical development of a fire is assumed (based on conditions in Reference 1). In this standard scenario the fire is assumed to grow without the intervention of any active fire safety measures. This fire is defined by several consecutive main stages, as illustrated in Figure 1 in technical level 1 below.
Typical stages of fire development (Technical level 1)
Initially, there is a pre-burning process eventually leading up to an ignition. Following the ignition, the fire starts to grow. The growth of the fire is mainly dependent on the availability and the characteristics of the fuel source. At one point, the temperatures or the radiation towards the floor in the room become so great that all available combustibles in the room ignite. This process is called flashover and is a rapid transition from a localized fire to a fully developed fire. During the fully developed fire stage the heat release rate is at its peak. The magnitude is dependent on the oxygen supply to the compartment. The fire at this stage is therefore described as a ventilation-controlled fire, in contrast to the pre-flashover conditions where the fire can be described as a fuel-controlled fire. The last stage of the fire is the decay stage, characterized by the combustibles in the compartment having been consumed to the point that the heat release rate cannot be sustained any further, with the result that the fire intensity starts to decrease [16].
Figure 1: Typical stages of fire development [1].
In all buildings, the movable items (furniture, equipment, goods, etc.) form the essential fire load at least for the early, developing phase of fire. The combustion process releases energy, gases and smoke. Toxic gases and smoke are the main cause of fatalities in a fire (approx. 80 % of fire fatalities are due to toxic fire effluents), while heat is the primary reason for damage to the structure of a building. As the mechanical and thermal properties of building materials change with increasing temperatures, knowledge of the time-temperature development during a fire in a building is most important for the structural fire analysis.
Fire load is defined as the energy (total heat release) of the combustible material present within the internal bounding surfaces of a room, compartment or building; a combination of the energy of the structural building elements and their contents. National regulations may also categorise building types according to expected fire load levels. In principle, fire protection by controlling the amount of fire load is possible, but in practice the difficulties depend on the energy of the contents of the building, which is mostly the main source of the fire load, and the magnitude of which is not controlled by regulations (only assumed categories may be used).
Furthermore, it is generally assumed that the structural elements themselves will not be directly involved in the fire, being protected from combustion by sacrificial linings (or in directly exposed timber by charring of the surface). As such, the energy from the structural elements is usually released much more slowly than energy from the contents of the building.
The ignition of timber building elements needs to be carefully considered, at the start of a fire but also throughout the fire exposure period. This needs to be controlled by two possible measures:
- Encapsulation of building elements (the method most often used), e.g. by non-combustible lining materials.
- Impregnation or coating of building elements with fireproof agents (analogous to wood preservatives). Treatment and working with fireproof impregnating and coating systems requires expert knowledge and must be carried out carefully. The long-term behaviour and durability of impregnating and coating systems should be considered, and is especially relevant for external wood building components.
Fire protection of timber by means of encapsulation of building elements is most common and has proven to be successful in most applications. The building elements and other combustible materials (e.g. insulation) are protected with fireproof layers that encapsulate the building elements and protect them from potential ignition. For more details in regard to wood products on surfaces refer to Reaction to fire performance – internal surfaces. Where timber is exposed directly, in all forms of timber construction but especially in mass timber applications, detailed review of performance characteristics is required to ensure fire safety objectives are met throughout the fire exposure.
Fire severity
To describe the fire action with simplified models, several nominal fire curves have been proposed in codes for use in the design process. Nominal fire curves provide a simple relationship for the temperature of the gases in a compartment as a function of time. However, they represent the fully developed fire phase, which means that the significant amount of time that sometimes elapses from the beginning of a fire until the fully developed fire phase is not included in the curves. In addition, the cooling or decay phase of the fire is not explicitly taken into account. (The effect of the cooling phase may, however, be taken into account implicitly by fire resistance requirements). The most frequently applied fire curve is the ISO 834 [2] standard fire curve, which is also used as the main exposure condition in European EN standards for fire resistance [3].
More realistic models of fires are delivered by parametric fire curves, which take into account the most important parameters for temperature development, namely:
- – the fire load (amount, type and arrangement of combustible material),
- – the ventilation conditions in the room,
- – the thermal properties of the enclosures, and
- – the fire fighting action.
Nominal temperature-time curves (Technical level 1)
For design by classification, nominal temperature-time curves are used for a specified period of time, without any cooling phase. The period of time, normally specified by national regulations, is assumed implicitly to take into account features such as risk assessment, measures of active fire protection and the cooling phase. Three nominal temperature-time curves are specified in the Eurocode (see EN 1991-1-2 [4] Clause 3.2, and Figure 2):
- Standard temperature-time curve
- External fire curve
- Hydrocarbon curve
For fire design of timber structures, it is mainly the standard temperature-time curve that has been used; and so almost all knowledge is based on this fire curve.
Figure 2: Nominal temperature-time curves as given in EN 1991-1-2 [4]
Parametric fire curves (Technical level 1)
As an alternative to nominal temperature-time curves, other fire models are used in the fire design of structures for temperature and structural analysis for the full duration of the fire, including the cooling phase.
A natural fire scenario describes the complete development of a fire, including the cooling or decay phase, as a relationship between the gas temperature in the fire compartment and time as a function of fire load density, ventilation conditions (opening factor) and thermal properties of the enclosing walls and floors: see EN 1995-1-2 [5] Annex A. Annex E of EN 1991-1-2 [4] gives the design value of the fire load density, dependent on various parameters such as the risk of fire activation due to the size of compartment and type of occupancy, as well as various measures of active fire protection, such as sprinklers, detection, automatic fire alarm or fire-fighting actions. The application of these parametric fire scenarios may be limited to specific European countries in accordance with relevant national annexes to EN 1991-1-2 [4].
Parametric fire curves can be used in building design utilising fire safety engineering. However, only limited information is available concerning the performance of timber structures in natural fires. Therefore, parametric fire curves are applicable to timber compartments only if the timber surfaces are encapsulated such that they do not become exposed during the fire and do not otherwise contribute fuel to the fire.
Fire safety design
To design a fire safety strategy you have to understand the behaviour of fire, buildings and their occupants and buildings during a fire. Fire safety strategies need to address life and property hazards in buildings and neighbourhoods, including environmental aspects. Chosen strategies may vary considerably, depending on such considerations as emphasis on passive or active fire protection, type and use of the building, etc.
To implement strategies, guidance on the tools to be used and how to apply them is needed. This guidance is given in national regulations, which present rules in terms of fire classes and numerical values to assess fire safety and/or performance requirements. In addition, tools for calculating such aspects as performance of structures (Eurocodes) and simulating the spread of fire and evacuation of occupants, are needed.
When designing fire safety in buildings, an understanding of fire safety objectives, the means of fire protection and the general concepts of evacuation safety will be required. The various means of designing building components, their installation and other protection measures will be complementary and key in achieving the fire safety objectives. This is the basis of the design solutions included in the fire website and applicable to all buildings. These topics are described further in the following sections.
How fire safety objectives link to design and fire management strategies (Technical level 1)
This diagram provides an example of how fire safety objectives link to design and fire management strategies, including building components, active and passive protection. National performance or threshold requirements can be introduced for various stages, the most commonly adopted are indicated below. In addition countries will regulate fire containment and suppression requirements.
Fire safety objectives
In European countries, the overarching principles forming the basis of fire safety regulations (both prescriptive and performance-based codes) of buildings are as follows:
- Occupants shall be able to leave the buildings or be rescued
- The safety of rescue teams shall be taken into account
- Load-bearing structures shall resist fire for the required minimum duration of time
- The generation and spread of fire and smoke shall be limited
- The spread of fire to neighbouring buildings shall be limited.
Note that the rescue of occupants may not always be a regulatory issue in all countries.
These principles have been formulated in order to be able to fulfil the following objectives:
- Safety of life
- Safety of occupants
- Safety of rescue teams
- Loss prevention
- Structures of building
- Contents of building
- Uninterrupted operation
- Public image
- Environmental protection
- Release of hazardous substances
Note that national regulations may concern only the first of these objectives, safety of life. In this regard the type of occupancy is of vital importance. It is used to determine the risk profile and forms the basis for the fire safety strategy for a building, bearing in mind that different occupancy types can be present within the same building.
Occupancy categories (Technical level 1)
Generally buildings are categorised in the following main occupancy types, also considering the use of the building:
- Single occupancy – a building containing a single occupancy and type of use (e.g. residential accommodation)
- Multi-occupancy – a building containing more than one occupancy and generally one type of use
- Mixed-use building – at least one other type of non-residential occupancy (e.g. shop)
The occupancy characteristics take into account whether occupants are likely to be familiar or unfamiliar with the building and whether they are likely to be awake or asleep.
A | Occupants who are awake and familiar with the building | Office and industrial premises |
B | Occupants who are awake and unfamiliar with the building | Shops, exhibitions, museums, leisure centres, other assembly buildings, etc. |
C | Occupants who are likely to be asleep | |
Ci | Long-term individual occupancy | Individual flats without 24h maintenance and management control on site |
Cii | Long-term managed occupancy | Serviced flats, halls of residence, sleeping areas of boarding schools |
Ciii | Short-term occupancy | Hotels |
D | Occupants receiving medical care | Hospitals, residential care facilities |
Table 1: Occupancy characteristic categories for different types of buildings
(Source: BS 9999:2017)
The different levels (concepts, objectives, strategies, systems and components) of establishing fire safety are illustrated in Figure 3.
Achieving absolute safety is impossible, regardless of the construction materials and systems involved. The level of acceptance must be quantified by the authorities or, with regard to financial losses, with the owner or the insurance companies.
These objectives can be achieved with different fire safety strategies that take into account the type and use of the building. Fire safety strategies consist of comprehensive structural (passive), technical (active) and organisational measures to achieve the predefined fire safety objectives and acceptance criteria.
The most efficient fire safety concept is arrived at by combining different options. The criteria for the evaluation of different options that fulfil the predefined fire safety objectives at the same level are the total cost of fire safety measures and further aspects such as flexibility, limitation of use, architectural design etc.
Different types of strategies are employed to meet these objectives. Tools available for use to prove/demonstrate the function of a building in the case of fire are briefly described in Figure 3.
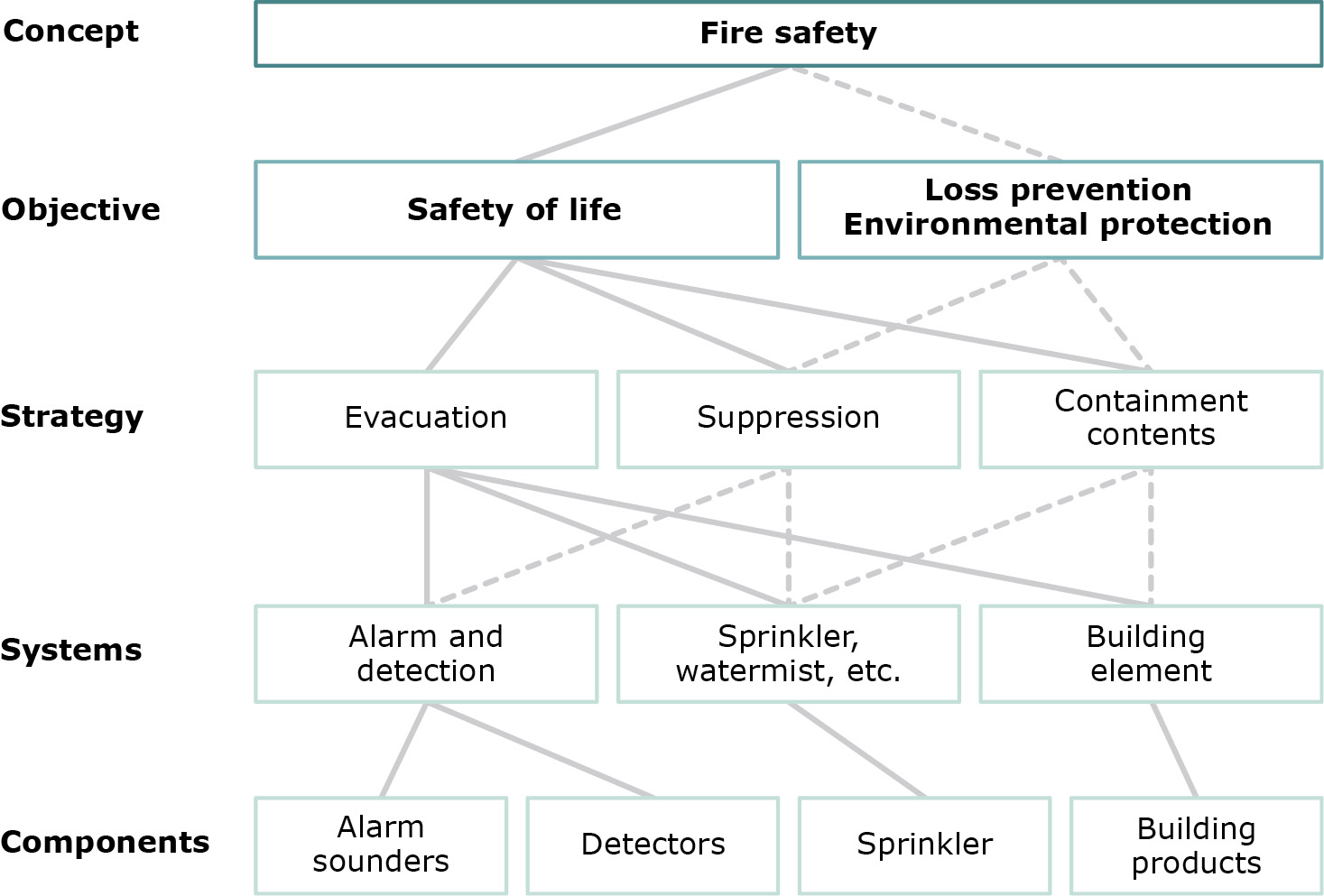
Figure 3: Fire safety from concepts to components.
Means of fire protection and evacuation safety
This site provides detailed design information on passive fire protection by providing guidance on the load-bearing and separating functions of timber structures under standard fire exposure, and by providing information on the effects of fire loads. However, active means of fire protection are also essential in fulfilling fire safety objectives, especially concerning the safety of life. The main means of passive and active protection are summarised in the following.
Structural (passive) fire protection is provided by building elements designed and constructed to maintain their load-bearing and/or separating function for the required minimum duration of fire exposure. Building elements with a separating function form the boundaries of fire compartments and must prevent the spread of fire to or from other parts of the building, or an adjoining building.
The generally employed principle of compartmentation is to subdivide buildings into areas of manageable risk, to give enough time for escape and rescue, and to provide fire separation for adjoining buildings. In a fully developed fire within the room of origin, the safety of occupants is no longer an issue, and a total loss of the contents is accepted.
A fire rarely spreads from one area of a building to another by destroying or burning through a wall or a floor. It usually spreads via common concealed spaces in walls or ceilings: heating, ventilation and air-conditioning duct systems, holes that have been cut through walls for electrical or communications cables, doors left open, etc. Thus, in order to ensure the integrity of a fire compartment, it is necessary that every opening or penetration through its walls, floor and ceiling, whether large openings, such as doorways or small service penetrations such as wiring, piping, and ducts, is protected to resist the spread of fire. Information on fire doors can be found here. Fire can also spread from the compartment through windows and along facades and/or to eaves and roof structures. Accurate detailing and quality assurance during construction are therefore essential for effective structural passive fire protection.
Fire resistance periods in national regulations generally range from 30 to 240 minutes (in increments of 30 minutes), depending on the height and use of the building, and the location of the building element concerned within the building. Traditionally, the fire resistance of an element has been determined by testing, i.e. by subjecting it to a ‘fire resistance’ test.
Fire resistance test (Technical level 1)
This is a furnace test that exposes a representative piece of structure to a prescribed heat exposure, with the building element being required to remain structurally load-bearing (stable), to shield heat (insulation) and to prevent the passage of smoke and hot gases (integrity). As an alternative to fire testing, verification by calculation is increasingly becoming more important: see, for example, EN 1995-1-2 [5]. However, there are no calculation methods for the integrity criterion.
In summary, passive fire protection measures include:
- Adequate compartmentation to inhibit the spread of heat, smoke and gases
- Limitation of fire compartment size
- Control of flammability/combustibility of wall linings – particularly on escape routes
- Control of the spread of smoke
- Provision of protected escape routes (information on fire doors can be found here).
- Provision of adequate thermal insulation, stability and structural performance
- Fire stopping.
The design of timber elements to provide a separating function and the prevention of fire spread within and through structures is described further in detail in Separating timber wall and floor assemblies and Cavity barriers and fire stops.
Active fire protection
As a complement to passive fire protection, active fire protection systems can be used to increase the fire safety level in buildings for special groups (safety of life), e.g. elderly or handicapped persons, for industrial or public buildings, or for fulfilling insurance requirements. Active fire protection systems can also be used in some cases as an alternative to some passive fire protection requirements.
Fire detection and alarm systems are used to provide means of detecting fire and giving warning. It is essential that an outbreak of fire be detected at an early phase so that the occupants are alerted and the emergency fire action plan can be implemented as soon as possible.
Portable fire extinguishers and hose reels can be used for initial fire-fighting, which can significantly reduce risks to persons if staff have been trained to use them.
Water sprinkler and high-pressure systems are widely used in the process industries for protection of storage vessels, process plant, loading installations and warehouses. The purpose of an automatic fire-fighting system is to extinguish the fire or at least control it until the fire service can take over. Particular attention is paid to the potential of residential sprinklers, i.e. simplified sprinkler systems connected to the tap water system.
Smoke extraction is used to improve visibility and reduce temperature. The simplest means are to use windows and doorways, but more effective results can be obtained by using special smoke vents, whether manual or automatic.
Effectiveness of fire service actions will depend on such factors as response time (dependent on distance and complexity of the site) and resources available.
In summary, active fire protection measures include:
- Provision of alarm systems – audible, visual etc.
- Provision of automatic fire detection
- Indication of the location of a fire on a visual display, mimic diagram etc.
- Provision of smoke/heat venting and exhaust control systems to extend escape time
- Closing of smoke dampers in ducts and closing of doors to create fire compartments etc.
- Release of access control systems on doors, gates, turnstiles etc. to facilitate escape
- Provision of automatic fire suppression systems.
The positive effect of active fire protection measures may be taken into account in the requirements. Means of active fire protection are described in more detail in Active fire protection.
Evacuation safety
The distinction between active and passive fire protection measures is not always clear-cut, as some measures are a combination of both, such as smoke control doors, which remain open under normal circumstances but are automatically closed on receiving a signal from a detecting device, or protective coatings, which intumesce and become effective only when a fire occurs. The doors and the coatings in these two cases remain part of the passive defence system, and it is only the detection and operating mechanism that is an active measure. Evacuation safety is also based on both passive and active elements. The main principles are briefly described below.
Emergency evacuation means the immediate and rapid movement of people away from the threat or actual occurrence of a hazard such as fire. Evacuation plans are developed to ensure safe and efficient evacuation of all occupants of a building. Proper planning will use multiple exits and alarm/guidance systems to ensure complete evacuation. These may include alarm signals that use both aural and visual alerts. Personal situations or factors that may affect an individual’s ability to evacuate must also be considered.
The main requirement is that safe evacuation of a building must be possible in the event of fire or other emergency. There must be a sufficient number of appropriately located exits that are sufficiently spacious, easily passable and clearly marked, so that the time to evacuate the building does not cause danger. The exits must lead outside to ground level or to a place that is safe in case of fire. It is key to maintain provisions for evacuation safety in service. When a building is in use, occupant behaviour is a key to achieving evacuation safety, escape routes can become blocked and evacuation discipline can be poor. Regular inspection and awareness of expected behaviour is key to achieving the fire safety measures.
Summarising, the following types of requirements exist in national regulations:
- The greatest permitted distances to the nearest exits are regulated at national levels
- Number of exits – the main principle is that there must be at least two separate, appropriately located, exits from each evacuation area
- Dimensions of exits – the minimum width and height are specified
- Fire-separation and construction of exits – an exit is often considered as a fire compartment of its own, and the use of combustible materials on surfaces is regulated
- In general, doors must open in the escape direction of the passage, must be easy to open in an emergency situation, and exits must be provided with emergency/exit lighting
- The exits and the access to them must be marked with symbol signs, and evacuation floor plans can be used to show exit routes.
Traditionally, the evacuation capacity of a building is designed according to a set of relatively simple design criteria (as described above) concerning the required width and length of evacuation routes, complemented by detailed requirements in the national building regulations.
Estimating evacuation time (Technical level 1)
The simplest methods for estimating evacuation time are manual calculation methods, in which pre-movement and movement times are taken from values in tables. However, the increased size and complexity of buildings make new demands on the methods used for fire and evacuation simulations. It would not, for example, be possible to build large multi-purpose centres using simple design rules. Instead, such places are usually designed using the performance-based design method, in which the safety of the design is studied as an entire system. The evacuation simulations must be able to model the dynamics of large and high-density crowds. The models should also consider the evacuees’ decision-making processes behind the reaction times, pre-movement times and the exit route selection during the actual evacuation [6] [7]. For an individual person, escape and evacuation from a building during a fire is always an extraordinary situation.
It is recommended that the design of safe evacuation of a building and the use of a defend-in-place strategy should be entrusted to professionals. They can determine the level of complexity of the method needed for the particular case and are capable of using the more advanced methods, if required.
Means of fulfilling fire safety objectives
There are a number of means of designing measures and features that, in combination, will deliver fire safety objectives. This is essentially independent of the type of materials and systems used, though detailed knowledge of performance characteristics, available options and user and building insights are key. Essentially two types of approaches are available:
- Prescriptive, where specific build or other solutions are provided and deemed to satisfy the fire safety objective(s)
- Performance-based or functional, where the expected performance is stated (for example in terms of a threshold for fire spread speed) and methods of assessing potential solutions are listed for the user to test their proposed solution against.
Prescriptive and performance-based approaches (Technical level 1)
Prescriptive fire regulations give detailed rules leading to an accepted standard, that is considered to give an acceptable level of fire safety, a concept of build, use of materials or other measures. The use of standard fire safety concepts does not require any further verification and, when built as outlined, guarantees acceptance by the fire authorities reviewing the application against requirements.
Performance-based, or functional, design concepts are more flexible and will allow other methods of proving compliance with requirements, for example, using detailed probabilistic and engineering-based analysis to optimise fire safety measures [8] [9]. This approach tends to be common in applications where standard, prescriptive solutions are not easily applied, for example in buildings of unusual shape or use and where only limited performance experience in the application is available.
National fire safety requirements will differ a lot in details and to some extent also in the expected safety levels. In most countries, there are two alternative ways of satisfying the national requirements:
- The building is designed and built in accordance with standard concepts (prescriptive design) by applying detailed rules with regard to fire classes and criteria provided by the regulations and guideline.
- The building is designed and built based on the application of science and engineering, taking into account specific characteristics of the building under construction and design fire scenarios, which must cover the conditions that are likely to occur in the building (performance-based design). This approach is used increasingly throughout the world.
Note that, at national levels, these principles may also be applied to parts of a building. In some countries (e.g. the UK) guidance on regulations specifies the level of performance expected, e.g. 60 minutes’ fire resistance, but does not prescribe ways of meeting this requirement. The guidance on regulations provides a ‘deemed-to-satisfy’ solution that provides this performance level, but the user can use other solutions if they so wish.
Prescriptive codes
The use of standard fire safety concepts as set out in prescriptive regulations does not require any further verification of the fire risk, and generally guarantees acceptance by the fire authorities (see Structural design approaches). For most small and medium-sized standard residential and office buildings it is cost-effective to base design on prescriptive fire safety solutions. Structural and product requirements are simply taken from tabulated values, and performance of the planned structures and products is taken from manufacturers’ data sheets, approvals and tabular values, or are calculated according to the Eurocodes (as modified by national Annexes).
Refer to Reaction to fire performance – internal surfaces for further details regarding the prescriptive codes.
Performance-based codes and design tools
A performance-based, functional approach to fire safety design relies on the use of fire safety engineering principles, calculations and/or appropriate modelling tools to satisfy building regulations. Building practitioners have flexibility in the application of fire safety by being able to choose the performance-based approach, the prescriptive approach or a combination of both. Fire safety engineering is becoming more critical as buildings get larger and/or risks for people and/or property increase.
The rapid development of modern building technology has resulted in unconventional structures and design solutions, with the physical size of buildings continually increasing. The interior design of many buildings with large light shafts, patios and covered atriums inside buildings connected to horizontal corridors or malls, introduces new risk factors concerning the spread of smoke and fire. Past experience or historical precedents (which form the basis of current prescriptive building codes and regulations) rarely provide the guidance necessary to deal with fire hazards in unconventional buildings [10] [11].
Instead of prescribing exactly which protective measures are required (such as prescribing a number of exits for evacuation purposes), the performance of the overall system is presented against a specified set of design objectives (such as stating that satisfactory escape must be effected in the event of fire). Fire and evacuation modelling, together with experimental evidence, is used to assess the effectiveness of the protective measures proposed in the fire safety design of a building.
Probabilistic and deterministic analysis (Technical level 1)
The linked acceptance criteria may be comparative as well as absolute. In using comparative criteria, the level of safety is based on prescriptive regulations, i.e. providing a solution that is considered ‘as safe’ as the prescriptive approach. The results of analyses are compared with the prescribed acceptable solutions. Absolute criteria are, for example, factors such as the threshold values for maximum heat flux and maximum temperatures to which humans could be exposed. Statistics, experimental data, experience, etc. are needed to determine the criteria.
Fire process models (Technical level 1)
More guidance on performance-based design and fire risk analysis is given in Structural design approaches.
Statistical analysis of fires in timber structures
One recent study, 2021, has been undertaken to attempt this, in part prompted by insurance questions regarding risks of property loss [12].
For this study, damage data of fire incidents in buildings constructed after 1995 from the USA, Canada, Sweden, New Zealand were analysed. The extent of fire damage or the financial damage was compared for fires in multi-storey buildings of timber construction types and fires in multi-storey buildings of other construction types. The study focused on property damage and property loss only. The risk of injury or death by fire was not considered.
In addition to the comparative study, a qualitative analysis of 33 high damage fire incidents in multi-storey timber buildings was also completed. The findings identified the most critical paths of fire spread, indicating most important detailing to improve property safety (see Cavity barriers and fire stops).
The presence of sprinklers and their impact on fire damage was also investigated. Further details can be found in Technical level 1 below.
The review focused on timber construction as a general term, noting that choice of technologies, type of engineered timber materials and construction methods can vary by country. To illustrate this point, statistics for Sweden were analysed. These show that approximately 9 out of 10 timber buildings in Sweden are wood/timber frame structures. (see figure 1), the remainder being constructed of CLT/mass timber. This ratio is expected to be significantly different in central Europe, for example, where mass timber is more popular.
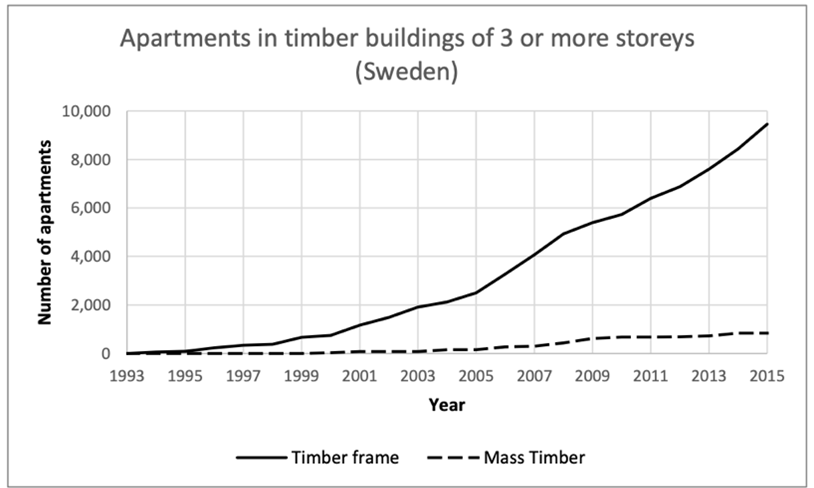
Figure 4: Number of apartments in buildings with a timber frame and mass timber construction. Determined from a database by RISE (Eriksson et al. 2016).
The study did not identify statistically significant difference between modern multi-storey buildings of timber and of other construction, noting that:
- – Lack of a statistically significant difference can be caused by lack of difference, or insufficient data.
- – As indicated by Garis and Clare (2014) the presence of sprinklers has a significant influence.[13]
Further findings (Technical level 1)
- No statistically significant difference between buildings of timber and other construction types was found in the data from the USA for buildings of 5 storeys or more. However, there are indications that the data for this category is unreliable.
- No statistically significant difference between buildings of timber and other construction types was found in the data from British Colombia (Canada) for buildings of 5 and 6 storeys. This holds for sprinklered and non-sprinklered buildings.
- A statistically significant difference between buildings of timber and other construction types was found in the data from British Colombia (Canada) for buildings of 1 to 4 storeys, without an automatic sprinkler system.
- No statistically significant difference between buildings of timber and other construction types was found in the data from the British Colombia (Canada) for buildings of 1 to 4 storeys, with an automatic sprinkler system.
- No statistically significant difference between buildings of timber and other construction types was found in the data from Sweden for buildings of 3 storeys or more. The data this is based on includes sprinklered and non-sprinklered buildings.
- In contrast with findings of previous research, analysis of the Swedish data indicated no difference in the frequency of fires in multi-storey buildings of timber and of other construction types over the same range of construction years.
- Buildings in New Zealand with timber frame structures of three storeys or more that were constructed after 1991 did not show a higher relative frequency of fire spread beyond the fire cell of origin.
- If old buildings, constructed until 1991, are included in the New Zealand analysis, the relative frequency of fire spread beyond the fire compartment of origin is higher for buildings with timber as the main structural material than for other types of buildings.
- Fires in modern timber buildings (constructed after 1991) in New Zealand generally resulted in less water damage than fires in other types of buildings.
Bibliography (Technical level 2)
1 Buchanan A H. Fire performance of timber construction. Progress in Structural Engineering and Materials, Volume 2, 2000.
2 ISO 834-1. Fire-Resistance Tests – Elements of Building Construction – Part 1: General Requirements. International Organization for Standardization, 1999.
3 EN 14135. Coverings — Determination of fire protection ability. European Committee for Standardization, Brussels, 2004.
4 EN 1991-1-2 Eurocode 1. Action on structures Part 1-2: General action – Actions on structures exposed to fire. European Committee for Standardization, Brussels, 2002.
5 prEN 1995-1-2 (2021) Eurocode 5. Final Draft. Design of timber structures. Part 1-2: General – Structural fire design. European draft standard. CEN European Committee for Standardization, Brussels.
6 Korhonen T, Hostikka S, Keski-Rahkonen O. A proposal for the goals and new techniques of modelling pedestrian evacuation in fires. Proceedings of the 8th International Symposium on Fire Safety Science. Beijing, China, 18 – 23 Sept. 2005. Gottuk D & Lattimer B (eds). International Association of Fire Safety Science, pp. 557 – 569.
7 Ehtamo H, Heliövaara S, Hostikka S, Korhonen T. Modeling Evacuees’ Exit Selection with Best Response Dynamics. Klingsch W. et al. (Eds.), Pedestrian and Evacuation Dynamics 2008 (The PED 2008 Conference Proceedings). Springer, pp. 309-319. The PED 2008 Conference in Wuppertal, Germany.
8 Fontana M, Maag T. Fire risk assessment based on Bayesian networks. Proceedings of the 5th International Conference on Performance Based Codes and Fire Safety Design Methods, Luxembourg, 6-8 October 2004, Society of Fire Protection Engineers, Bethesda, MD, USA.
9 Joyeux D, Bonnot S, Hietaniemi J, Korhonen T. Risk-based fire safety engineering approach applied to a public building. Proceedings of the 5th International Conference on Performance Based Codes and Fire Safety Design Methods, Luxembourg, 6-8 October 2004, Society of Fire Protection Engineers, Bethesda, MD, USA.
10 Thureson P, Sundström B, Mikkola E, Bluhme D, Steen Hansen A & Karlsson B. The use of fire classification in the Nordic countries – Proposals for harmonisation. SP Technical Research Institute of Sweden. SP Report 2008:29, 2008.
11 Dehne M, Pape H, Kruse D, Krolak M. Brandschutzkonzepte für mehrgeschossige Gebäude und Aufstockungen. Informationsdienst Holz, Bonn, 2005.
12 SAFITS Statistical Analysis of Fires in Timber Structures Brandon, D., Plathner, F.V., Mikkola, E.
13 Garis, L and Clare, J, Fire Outcomes by General Construction Type A Retrospective Analysis of British Columbia Reported Fires, February 2014
Other useful references
EN 13501-2. Fire classification of construction products and building elements – Part 2: classification using data from fire resistance tests, excluding ventilation services. European Committee for Standardization, Brussels, 2007.
Hadvig S. Charring of wood in building fires. Technical University of Denmark, Lyngby, 1981.
Toft Hansen F, Bolonius Olesen F. Full-scale tests on loaded glulam beams exposed to natural fires. Department of Building Technology and Structural Engineering, Aalborg University, Aalborg, Denmark, 1992.
König J. Effective thermal actions and thermal properties of timber members in natural fires. Fire and Materials, 30:51-63, 2006.
Fontana M, Maag T. Fire risk assessment based on Bayesian networks. Proceedings of the 5th International Conference on Performance Based Codes and Fire Safety Design Methods, Luxembourg, 6-8 October 2004, Society of Fire Protection Engineers, Bethesda, MD, USA.
Staffansson, L. Selecting Design Fires. Lund: Lund University, Sweden, 2010.
RISE Report :2021:97 ISBN:978-91-89385-87-0 Borås, 2021
Fire safety in timber buildings Technical guideline for Europe. Main results from the WoodWisdom-Net Research project FireInTimber – Fire resistance of Innovative Timber structures
Östman, B and Buchanan, A, Fire Safe Use of Wood in Buildings. Global Design Guide (DOI: 10.1201/9781003190318’)